Buildings
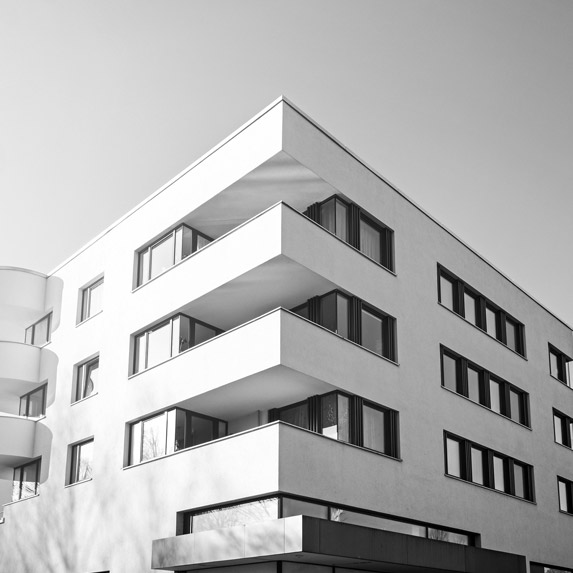
Buildings represent an estimated 36% of global final energy consumption and 39% of the global energy-related greenhouse gas (GHG) emissions.[1] The goal of total decarbonization in the buildings sector includes the construction of new buildings and districts with zero or almost zero energy consumption from fossil fuels and the total renovation of existing buildings with the same net zero carbon standards. Current renovation rates account for about 1% of existing building stock each year, while to achieve 100% zero carbon goal by 2050 it is necessary to ensure a renovation rate higher than 3%.
It should be noted that the CO2 emissions resulting from material use in buildings represents almost one third of building-related emissions: the construction industry must radically change its manufacturing structure in order to abate this increasing embodied energy.
In general, using a combination of readily available technologies and approaches, and performance-based design metrics, net zero carbon buildings and districts can be achieved today, according to the following strategy:
- maximize the buildings energy efficiency first, mainly through passive and low embodied-carbon solutions;
- Adopt high-efficiency technical systems and advanced control/management strategies: the phase out inefficient solutions, encouraging of low-carbon systems such as heat pumps and district heating and the adoption of advanced control/management strategies, is the second priority.
- Maximize on-site or nearby renewable energy production and self-consumption while electrifying the buildings sector, to completely cover or exceed the total energy demand of each building with the minimum exchange of energy with the grid (thus stimulating energy management, storage and exchange at district level).
This will result in different combinations of solutions that are appropriate for each specific context, obtaining buildings that are resilient to climate change effects. Moreover, in order to achieve the overall decarbonization of the buildings sector, energy consumption related to cooking must also be addressed.
The action areas in this sector include:
- Establish advanced building energy codes with mandatory performance standards and set minimum energy performance levels for existing buildings. Policies and subsidies to favor the retrofit of existing buildings rather than new constructions are absolutely necessary.
- Achieve high efficiency building envelopes at negative life-cycle cost, mandate energy performance standards for envelope components and work with industry to deliver non-invasive and whole-building retrofit packages. Policy makers should develop strategic frameworks to create the adequate market conditions for low-carbon technologies, guiding building owners and designers in making the correct choices.
- Mandate minimum energy performance standards for stand-alone heating equipment, prevent expansion of fossil fuel heating and pursue strategy to shift demand to high efficiency and integrated energy solutions with net zero emissions.
- Pursue low-cost solar cooling technologies such as high efficiency and renewable district cooling where appropriate. Mandate use of waste heat from large-scale cooling for heating and hot water use on-site or via district systems; local governments are uniquely positioned to advance district energy systems in their various capacities.
- Regulations and measures obstructing energy self-consumption such as specific additional taxes or levies should be lifted and administrative procedures to allow self-consumption should be user-friendly. • Achieve affordable thermal storage and low cost solar thermal systems (for low-income countries only).
- Training and capacity building activities for the construction sector must be adequately promoted, while also pushing the development of specific DSS (decision support system) or design-aid tools to strongly increase the application of climate-responsive and integrated building design.
Case Studies
Representative examples of low carbon districts/buildings for specific climatic contexts (i.e. cold and hot) are described below.
Schlierberg Solar Estate, Freiburg, Germany
“Am Schlierberg” in Freiburg is one of the first solar and energy-plus housing estates in the world.[2][3][4] The housing development was built in 1997 and has been acting as a trend-setting pilot project for solar building and clean energy living ever since.
The solar installation consists of 445 kWp grid-connected PV and string inverters mounted underneath the roof deck. The total annual solar electricity production is 420,000 kWh. The housing development was designed to assure optimum efficiency. Therefore, unobstructed solar radiation on the roofs throughout the year, summer shade, and winter sun on the southern façades were taken into account, and all south facing roofs were covered with horizontal area PV modules. This, together with the energy efficient building design, allows for 2 million kWh primary energy savings per year. Any excess generated electricity, which is not used by the residents, is fed into the public grid in return for a feed-in compensation, covered under the German National Renewable Energy Acts’ feed-in tariff.
Heat energy is generated by a local network of solar hot water evacuated-tubes, which are located on the “Sonnenschiff”, a 125 m service block. Any additional energy required during the winter months is provided by a local combined heat and power plant, fueled by wood chips and natural gas.The building’s low energy demand derives from a group of measures, such as the building design in compliance with the passive house standard, the high insulation standard, the average U-value for the building envelope (0.28 W/m2K), and efficient ventilation heat recovery. Additionally, the complex uses electricity-saving appliances and energy conservation practices.
The project’s aim is to apply German Passive House and Plus Energy House directives, as well as show sustainable construction principles through material selection, appliance choice, energy consumption, transportation options, and construction method. The project shows how the complex relation between a building’s location, orientation, layout, use, energy production and consumption can create a positive impact by generating energy and feeding it into the grid.
Higashimatsushima City, Smart Eco-Town, Japan
In the aftermath of the 2011 earthquake and tsunami, the Japanese city of Higashimatsushima experienced the worst flood damages of all cities in Miyagi prefecture. To establish a more sustainable and resilient energy infrastructure, the city of Higashimatsushima set a goal to become a net zero energy city by 2022.[5] The plan includes the creation of an experimental 85-home microgrid community, supplied by solar, wind, and biodiesel technologies, along with large-scale energy storage.
The community consists of 70 detached, single-family homes and 15 multifamily apartment buildings. The energy needs of the micro community will be met by solar (470 kW) and bio-diesel (500 kW) energy systems, and 500 kWh of energy storage to meet the entire town’s energy need of three days.
The town’s electrical grid infrastructure is developed and owned by the city, which operates as a regulated monopoly. By having its own distribution system, the city will get an electricity supply contract with either the regional utility or renewable energy independent power producers (IPPs), and distribute electricity to all 85 households.
The city will further apply a Community Energy Management System (CEMS). It will allow the city to monitor electricity consumption and generation data via individual smart meters, manage the energy storage system during peak-demands, and bill customers. During emergencies, the system will start the biodiesel generator to help control and balance the energy needs usually provided by solar and energy storage. This microgrid community is a joint project with the city of Higashimatsushima and Sekisui House, Japan’s leading housing developer, who received research funding from the Ministry of Environment.
Enerpos, Reunion Islands
ENERPOS (French acronym for POSitive ENERgy),[6][7] a two-storey university building, is located in a tropical climate. The building is split into two parallel wings and separated by a vegetated patio, with a total gross floor area of 739 m2.
ENERPOS applies a number of passive design strategies (mainly cross ventilation and sun-shading devices for the envelope) with the use of PV (covering the building’s peak demand for cooling) to reduce mismatch losses. The passive design strategies for cooling include the following: a 3 m band of native plants surrounding the building, the north and south orientation of the main façades (which are perpendicular to the thermal breezes that blow during the hot season), the building’s natural cross ventilation through its window to wall ratio (WWR) of 30%, and the use of external shading, (which allows for airflow regulation, while also providing protection against sun, cyclones and break-ins). Further, high-performance ceiling fans were installed to guarantee additional air speed during windless days. This allows for a transitional period before the use of active air-conditioning systems become necessary. Another cooling measure was the insulation of the roof with a 10 cm layer of polystyrene (less than 0.5% of the solar radiation comes through the roof).
Due to a proper design, the building’s electrical consumption from May 2010 to April 2011 was 9,824 kWh kWh/m2y. The main sources of energy consumption were the electrical outlets (46%), the split system used to cool the two technical rooms (15%), interior lighting (14%), ceiling fans (11%), the exterior lighting (7%) and the lift (7%).
The building’s photovoltaic roof covers an area of 350 m2 and enables the production of about 70’000 kWh/y of electricity. The building is a positive energy building as it produces more electricity than it consumes. ENERPOS, was built in 2008, with gross costs per square foot of about $111 and net costs per floor area of $232. The building shows that with current technologies and a small additional cost, it is possible to build a nearly zero-energy building (nZEB), even in hot climates.
Umoji House, Dar es Salaam, Tanzania
Umoja House[8][9] is considered a fitting response to the city of Dar es Salaam and its climate. The company BDP designed the structural and environmental engineering for the building. Today, the British High Commission, the embassies of Germany and the Netherlands, and the European Commission are located in the building.
The building consists of two blocks of office accommodation, which are supported by pilotis, connected by bridges and accessible by a central stair and lift. The buildings’ design incorporated considerations to protect the building from negative climate impacts. To prevent overall heat gain, the building has a floating solar roof and external louvres on three of its elevations. The building’s orientation is designed in respect of the sun’s movement. The longer facades have a north-south orientation, while the shorter facades face east and west to avoid the morning and evening sun. The facades are shaded to counteract increases in indoor temperatures. The building has two main wings with an open central bay linked to corridors and staircases. The site and courtyard are landscaped with local plants and trees which provide cool and comfortable space for public events and reduce the demands for cooling through natural ventilation and the thermal stack effect.
The glazed surfaces in all facades are protected by shading devices. These are comprised of stainless steel screens, which both shade the building and maximize the comfort of the users. In this climate, solar control devices are crucial in order to avoid excessive thermal gains. The Heating, Ventilation and Air Conditioning (HVAC) system is connected to solar collectors and bore hole water. It has a rainwater harvesting system that collects water in the basement, which is used for cleaning the building and for irrigating the grass. Construction materials were sourced locally wherever possible, and supported via local agents and contractors. The total construction cost of the building was £2.5m.
Global Alliance for Buildings and Construction (GLOBAC), United Nations Environment and International Energy Agency. “Towards a Zero-Emission, Efficient and Resilient Buildings and Construction Sector.” GLOBAC. Published 2018 ↩︎
UN-Habitat “Energy and Resource Efficient Urban Neighborhood Design Principles in Tropical Countries - A Practitioner’s Guidebook.” UN-Habitat (2018). ↩︎
PV Upscale. “ Solarsiedlung am Schlierberg, Freiburg (Breisgau), Germany.” PV Upscale. Accessed August 22, 2019. http://www.pvupscale.org/IMG/pdf/Schlierberg.pdf ↩︎
Department of Indoor Environmental and Building Services Engineering. “Solar Settlement Freiburg, Germany.” Czech Technical University in Prague. Accessed August 22, 2019. http://tzb.fsv.cvut.cz/projects/resset/files/resset-germany.pdf ↩︎
Born from Disaster: Japan Establishes First Microgrid Community, Junko Movellan 2015, Sekisuihouse. “Promoting Net-Zero-Energy Housing.” Sekisuihouse (2015). https://www.sekisuihouse.co.jp/english/sr/datail/__icsFiles/afieldfile/2015/08/19/P21-P52.pdf ↩︎
Lenoir, Aurelie and Francios Gard. “Tropical NZEB.” High Performing Buildings. Published 2012. http://www.hpbmagazine.org/attachments/article/11967/12Su-University-of-La-Reunion-ENERPOS-Saint-Pierre-La-Reunion-France.pdf ↩︎
UN-Habitat. “Sustainable Building Design for Tropical Climates.” UN-Habitat (2015). ↩︎
Zaha Hadid Architects, Gehry Partners, Foster and Partners, Rafael Moneo and more. Contemporary Architecture:CA2. Victoria: The Images Publishing Group Pty Ltd., 2004 ↩︎
UN-Habitat. “Sustainable Building Design for Tropical Climates.” UN-Habitat (2015) ↩︎