Industry
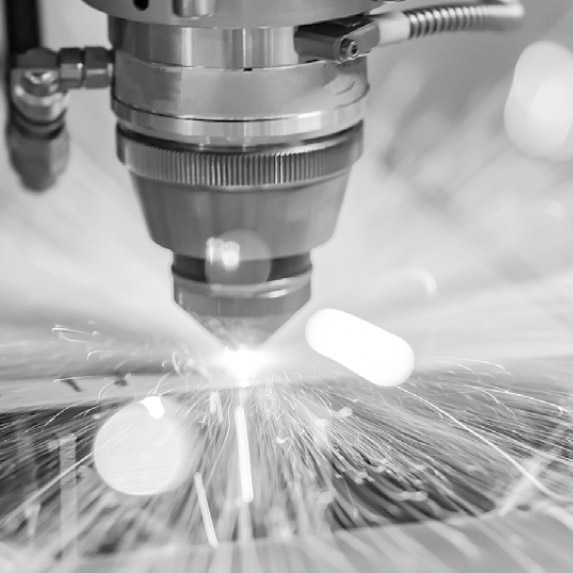
Heavy industry emits a large share of global greenhouse gas emissions, because industrial processes employ high temperatures and depend on high energy densities to enable the chemical processes involved. The industrial sector of the worldwide economy consumed more than half (55%) of all delivered energy in 2018. Within the industrial sector, the chemicals industry is one of the largest energy users, accounting for 12% of global industrial energy use.[1] Three energy intensive sectors are considered in this roadmap: cement, iron and steel, and petrochemicals (plastics, solvents, industrial chemicals) which each contribute to emissions of different kinds: i) direct, thermal, ii) direct, chemical and iii) indirect.
Emissions from these types of industries are significant and require solutions that go beyond electrification of the energy inputs, actually adjusting the chemical and physical processes associated with typical huge industrial plants operations. To reduce emissions, these industry sectors will need to displace fossil fuel-based energy inputs with low- to zero-emission electricity, along with improved heat integration and energy efficiency, all by taking advantage of new processes. Fully decarbonizing such complicated and integrated industrial environments requires a multidimensional approach.
Three action areas in this sector include:
- reducing demand for carbon-intensive products and services
- improving energy efficiency in current production processes
- deploying decarbonization technologies across all industries, which in turn can be split between four supply-side decarbonization routes
Electrification | Use of biomass | Use of hydrogen
and synthetic fuels | Use of carbon capture technology
There are currently no purely technological limitations blocking major decarbonization routes across any industrial sector. The barriers are economic and not technological; we have the technologies today but they are expensive. Moreover, the complexity of very integrated production plants introduces an additional obstacle: secondary products are used as feedstock for other plant sections. As a major consequence, changes in one process need to be introduced to ensure compatibility with the other processes.
Some material efficiency options for the three industrials sectors analyzed include:
- Cement: building design optimization, concrete reuse, materials substitution
- Iron and Steel: optimization of scrap recycling, product design for efficiency, more intensive use of products
- Petrochemicals: chemical and mechanical recycling, plastic demand behaviour change, use of renewable feedstocks, and product eco-design to better enable recycling
For these industries, improvements in energy efficiency should run in parallel with material efficiency and demand reduction. Appropriate technology for energy efficiency exists today and it can be applied in any country. Some of the key solutions for energy efficiency improvement include:
- Cement: switch to dry kilns, multistage cyclone heaters
- Iron and Steel: re-use of high-pressure gas for power, coke dry quenching
- Petrochemicals: energy efficiency in monomer production, and naphtha catalytic cracking
These solutions may result in a meaningful reduction in emissions, but policymakers must also promote change through economic and policy incentives.
Of course, also geographical contexts will impact technology decision making. Countries investing in new plants should go for zero-carbon technology rather than invest in energy efficiency improvements in plants at the end of their life. In contrast, countries where legacy plants and facilities will continue to operate for years to come should invest in energy conservation and energy efficiency improvements for existing processes. Additionally, the possibility of combining more of these solutions in a given country or facility will vary and depend on the geographical distribution of resources and social acceptability of specific technologies.
The pace at which technological breakthroughs are adopted will determine deployment opportunities over the next decades. Specific geographic and political scenarios play a critical role in the pace of technology development, adoption, and deployment, notably:
- the existence of appropriate policy incentives as well as other policy instruments
- reduction in the cost of alternative zero-carbon fuels, in particular zero-carbon electricity
- trade-exposed industries facing international competition from jurisdictions that have chosen to reduce their emissions at a slower pace
Case Studies
An example of the use of CCS technology with an industrial process is demonstrated at scale with the Quest Carbon Capture and Storage project in Alberta Canada where the CO2 emissions (about1MTonne/year) from a hydrogen manufacturing plant are captured, compressed to supercritical conditions, and then permanently sequestered in a saline aquifer about 900 meters below the surface.
Although the Quest project is being used to reduce emissions from an oil sands project, this case demonstrates that the technology is currently available. Effective carbon pricing and/or regulations are required for significant application to other heavy industrial applications.
Sendich, Elizabeth. “Today In Energy -Energy products are key inputs to global chemicals industry.” U.S.Energy Information Administration (blog). June 21, 2019. Accessed August 21, 2019. https://www.eia.gov/todayinenergy/detail.php?id=39952&src=email ↩︎