Executive Summary
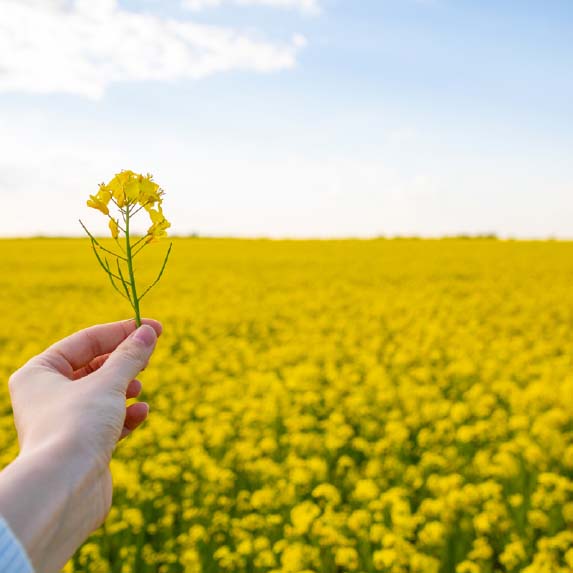
2020 marked a watershed year for nations to increase their emission commitments through the submission of updated nationally determined commitments (NDCs) as outlined in the Paris Agreement. As of early 2021, 124 countries accounting for 61% of global GHG emissions, have communicated a net-zero targets.[1] These commitments, the most ambitious the world has ever seen, will only be feasibly met if near term actions align with the long-term pathways needed to collectively halt emissions and balance the planet's carbon budget.
Accompanying many of these NDCs are Low-Emission Development Strategies (LEDs) which articulate the key sectors and technologies on which nations will rely to decarbonize their economies. In addition to these national commitments, companies and key sectors are also taking seriously the goal of net zero emissions for example by joining the Race to Zero campaign launched by Champions Gonzalo Muñoz and Nigel Topping. These commitments have been supported by a growing number of sector strategies, coalitions and roadmaps articulating their course to net zero emissions. In the transport industry, the Global Maritime Forum launched the Getting to Zero Coalition, Deloitte and Shell released a roadmap for decarbonising the global road freight industry and the International Civil Aviation Organisation (ICAO) are working on their Zero Climate Pact. While some of these commitments still fall short of the necessary ambition needed to keep warming to 1.5 C, they do indicate a shift in the historic rhetoric. Key to the success of any of these efforts is the successful scaling of the solutions employed to meet these goals. The following report will try to better understand the feasibility of scaling biofuels to replace liquid fossil fuels in emissions reduction efforts, with a focus on the impacts to land, water and local economies.
In these efforts to design pathways toward net zero emissions, the precautionary principle reminds us to carefully assess the sustainability and scalability of our solutions. The Getting to Zero 2030 Coalition has provided a high level description of the energy inputs it considers under the definition of a zero carbon fuels source. These include primarily biomass derived fuels, hydrogen and synthetic non-carbon fuels (ammonia) and synthetic fossil fuels.[2] However they clarify that "Fuels derived from biomass are another option for reducing GHG emissions. In terms of carbon accountancy, this is more commonly described as "net-zero" because biomass derived energy is normally still a hydrocarbon that on combustion releases CO2. But because the production of biomass takes CO2 out of the atmosphere in equivalent quantity to that emitted in combustion, it can theoretically be considered as net-zero. GHG emitted in upstream processes (e.g. land-use, harvesting, processing/refining, transport) needs to be accounted for in addition and currently results in a small net positive carbon emission." Some cellulosic biofuel pathways under development, especially those coupled with geologic Carbon Capture and Storage (CCS) have the potential to be a strongly carbon negative fuel and offset positive emissions. With a stagnant growth of the global biofuel supply chain, coupled with their inclusion in many of the transport industries long-term decarbonization strategies, the sector still begs the question: are biofuels sustainable and if so, at what scale and what role do they play in the future global energy mix?
Given the growing interconnectedness of our world, and the elaborate and complicated supply chains on which many of our goods and services rely, an integrated approach is needed to assess the life cycle performance of the solutions chosen to meet Paris Agreement emissions goals, ensuring that other delicate systems are balanced along the way, including socio-economic wellbeing of local communities and the environmental well being of the planets natural habitats. It is with this realization that this project has been undertaken as a joint research project by the UN Sustainable Development Solutions Network (SDSN) and the Fondazione Eni Enrico Mattei (FEEM).
Calling on the expertise of land-use, energy, water, and biofuel technology scientists, engineers and professionals from around the world, these partners convened a world class consortium to discuss, debate, and refine the potential for biofuels to be incorporated into national and sectoral decarbonization strategies. In the chapters ahead, these researchers attempt to better understand the intricacies of the global biofuel market, the evolution of key technologies, and the intersection of biofuel production on the land, water, and the local economies from which they are created.
As commitments from national governments require a progressive phase-out of fossil fuels as a primary source of energy in the next decades, this report intends to explore the potential role that biofuels can play to accelerate that process by analysing main production trends and emerging technologies. In addition, the report attempts to assess crucial controversies connected to their scalability, such as their impact on the environment and the potential competition of biofuel demands on agriculture and food production (in particular for traditional biofuels) in a global context characterized by increasing energy and food demand.
As per consolidated tradition, the approach adopted for such analysis is as holistic and inclusive as possible. The analysis carried out by authors and experts who took part in the drafting process of the Roadmap is diversified and offers perspectives according to specific local, national and regional contexts, as well as to specific economic sectors and technologies.
Biofuels' role in the global energy mix is still quite marginal. However, breakthrough technologies and management practices, including responsibly managed first generation biofuels, cellulosic bioethanol, advanced bio-oils, biogas from wastes, thermochemical processes, and synthetic biofuels, combined with the utilization of alternative feedstocks such as forest, wood, and agricultural residues; industrial and municipal wastes; and algae may raise their status as a valuable solution to tackle CO2 emissions for specific sectors -- notably long-haul shipping and aviation -- and in specific geographic contexts. Perennial biomass crops sequester carbon in the soil, and most biofuel conversion technologies produce nearly pure streams of waste CO2 containing 30% to 50% of the carbon in the original biomass; both of these mechanisms may justify a larger role for biofuels in addressing the expected overshoot of fossil emissions beyond the Race to Zero targets. The report's analysis spans from an assessment of biofuels' feedstocks sustainability -- ranging from traditional crop-based biofuels to second- and third-generation biofuels.
There exist important considerations in evaluating sustainability of biofuels, as biofuel production has had far reaching impacts for food, water, land use, and social systems. Biofuel production, especially first generation biofuels, can divert crops away from food production if not properly managed, causing decreased availability of and access to food, and decreased resiliency of food supply to shocks. Demand for biofuels may cause pastures to be converted into biofuel crop land, potentially resulting in deforestation to make space for new pastures; further as land cover changes and biofuel crops replace other ecosystems this can reduce natural carbon sinks. Additionally, using water for biofuel crops may put pressure on already-limited water resources. And finally, biofuel production can result in small-scale traditional farming being replaced by large-scale industrialized commercial agriculture, impacting social systems, cultural values, and economic opportunities and risking loss of traditional ecological knowledge, threatening exploitation and dispossession of cooperatively owned land.
Case studies of biofuels in Malaysia and Indonesia, Brazil, the United States, and the European Union put the findings of this report in context. In Malaysia and Indonesia, palm oil is a major export and production generates substantial revenue. Ensuring sustainability of palm oil production, and the potential for palm-oil based biofuels, will require diversifying downstream production possibilities; use of low-carbon land resources for production expansion; and holistic thinking that takes into account land, energy, and food and is creative about how multiple types of land use can co-exist.
In Brazil, all Brazilian vehicles use some type of biofuel, and bioenergy is the most important renewable energy source in the country. Local production of biofuels, mainly from sugarcane crops, has allowed for reduced energy imports, increased energy security, and brought social and environmental benefits. Brazil's success in biofuel use highlights the importance of R&D, which allowed Brazil to balance some of the water and land use challenges of biofuel production, and expanded the potential for biofuel use to be an effective solution for decarbonising transport.
U.S. biofuel use has faced varying public and government support; compounded by commercialization challenges and a "food-versus-fuel" debate, biofuel use in the U.S. has lagged in investment and uptake in comparison to other renewables. However, biofuel use for aviation, renewable natural gas, geologic carbon storage, and the potential of cellulosic biofuel crops to offer carbon mitigation along with biodiversity, water quality, and other ecosystem service benefits presents expanding opportunities for biofuel use in the U.S.
Currently the largest producer of biodiesel worldwide and among the top producers of advanced biodiesel/HVO, the EU's high level of production is supported by a strong policy framework. The EU's experience with biofuels illustrates their high potential connected to the mobilization and conversion of waste and residues, from both an economic and resource standpoint.
The authors of this report were challenged to answer the intricate question of are biofuels sustainable and if so, what criteria needs to be met in order to achieve sustainability on a global scale. Given the diversity of geographic and subject matter expertise included in this project, no single answer was found to address this question.
However, building off of work previously conducted and incorporating some of the latest research on the land- energy-water nexus of biofuels, the authors found that:
-
Historically, traditional biofuel production has been fraught with challenges related to land use competition with food resources, water scarcity issues, environmental degradation, and adverse impacts on global markets. Learning from these experiences and ensuring all spillover impacts from biofuel cultivation are addressed is key. In this report we highlight new technologies, practices in diversifying crop management, life-cycle analysis, and biofuel certification schemes that offer an opportunity to produce biofuels more sustainability in the future for the purpose of global and local emissions reduction efforts as they are used to replace fossil fuels.
-
Biofuels will never and should not be expected to produce 100% of our renewable energy needs, but 10% or even 20% globally is a feasible, realistic, and affordable path toward large scale negative emissions in the coming two decades.[3] This point is further substantiated by the International Energy Agency's Net Zero By 2050 analysis which states that "Low-emissions fuels today account for just 1% of global final energy demand, a share that increases to 20% in 2050 in the NZE. Liquid biofuels meet 14% of global transport energy demand in 2050, up from 4% in 2020; hydrogen-based fuels meet a further 28% of transport energy needs by 2050."
-
There are potential applications for biofuels in the pathways to a net-zero future, specifically in aviation, heavy- duty transport, and shipping, as long as a proper life-cycle analysis is completed in the production and supply chain of the fuel.
-
There is currently no globally optimal biofuel technology on the market. Optimal biofuel technologies and feedstocks must be assessed based on local contexts taking into consideration local socio-economic and environmental factors.
-
There are currently an array of certification schemes in place that can be used to assess the sustainability of biofuels and these should be built upon if the industry is to scale sustainably into the future.
-
Many traditional biofuel feedstocks compete with agricultural productivity, especially when land and water resources are constrained. The global biofuel market should be monitored and regulated to minimize these impacts and negate any impacts on food security.
Key Contextual Definitions
Biodiesel a renewable, biodegradable fuel manufactured from vegetable oils, animal fats, or recycled restaurant greases that undergo transesterification
Ethyl-tertiary-butyl ether (ETBE) a fuel ether, or blending component for fuel, that contains oxygen in a chain of carbon and hydrogen atoms, ETBE can be blended with gasoline or biofuels to decrease emissions and improve fuel performance because of its high oxygen and octane content. ETBE can be produced from ethanol and isobutylene (non-renewable), or through renewable ethanol and renewable isobutene (renewable)
Crop-based biofuels biofuels made from agricultural products, including sugarcane, wheat, corn, and soybean
Second generation biofuels biofuels produced from biomass sources such as wood, organic waste, food waste, and specific crops
Third generation biofuels biofuels produced from crops specifically intended for biofuels such as algae
Bioenergy renewable energy produced by living organisms
Pasture a land use type used for livestock grazing; may be cultivated and consists of vegetation such as grasses, legumes, other forbs, or shrubs
Rangeland lands where the indigenous vegetation is primarily managed through ecological versus agronomic processes; vegetation includes grasses, grass-like plants, forbs, and possibly shrubs or dispersed trees and spans grasslands, savannas, shrublands, most deserts, tundra, alpine communities, marshes and meadows
Marginal land land found on the edges of areas that are cultivated and is land that can be economically marginal (hard to make money on), biophysically marginal (hard to grow crops on), and/or socially marginal
Hydrotreated vegetable oil (HVO) straight chain paraffinic hydrocarbons produced through hydroprocessing of oils and fats; HVO is a diesel-type hydrocarbon that can be used as a substitute for diesel
Black, R., Cullen, K., Fay, B., Hale, T., Lang, J., Mahmood, S., Smith, S.M. 2021. Taking Stock: A global assessment of net zero targets, Energy & Climate Intelligence Unit and Oxford Net Zero. ↩︎
2021 Globalmaritimeforum.org. https://www.globalmaritimeforum.org/content/2019/09/Getting-to-Zero-Coalition_Zero-carbon-energy-sources.pdf. ↩︎
Haberl, Halmut, Karl-Heinz Erb1, Fridolin Krausmann1, Steve Running2, Timothy D Searchinger3 and W Kolby Smith. 2013. Environ. Res. Lett. 8 031004. ↩︎